Gallery / Research Groups
NCCR covers a broad range of research within the field of Quantum Science and Technology. Here you will find some impressions; for further information please visit the homepage of the relevant research group.
Research Groups
The core of a magnetic resonance force microscope consisting of a microcantilever, a laser interferometer, and a chip with radiofrequency antenna. In high vacuum and at cryogenic temperatures, the cantilever detects nuclear spins with nanometer spatial resolution.
Triple dot containing three electrons with well-defined spin directions coupled to the microwave electric field confined in a superconducting microwave resonator. The experiment demonstrates the strong coupling of a spin-qubit to a photon.
A purpose-made glass cell for experimenting with atomic gases at temperatures of less than 500 nanokelvin above absolute zero. These 'ultracold' gases are versatile quantum systems that can be controlled and manipulated with very high precision.
A self-assembled germanium hut structure, 2.5 nm in height, resides on a silicon surface. Structures of this kind serve as basic components for quantum devices.
Collective modes of billions of neodymium ions embedded in two optical crystals (here illuminated by red and green lasers, respectively) share quantum entanglement. Entanglement is a necessary requirement for quantum memories, which will be at the core of future quantum networks.
A structure made of several gold layers deposited on an alumina wafer serves as a trap for single ions. Particles trapped in such a manner can be controlled with a high degree of fidelity and provide versatile building blocks for quantum-information processors.
Topological phonon systems can be engineered in mechanical metamaterials where their stable surface modes can used for applications such as vibration isolation or reliable waveguides.
Collective excitations of a strongly correlated electron liquid give rise to a wealth of physical phenomena. By hybridizing such systems with light, we engineer novel quantum states to explore possibilities to manipulate photons using many body states of matter and contrariwise.
Parallel-plate capacitor with a suspended top plate that can mechanically oscillate. The aluminium capacitor is integrated in a superconducting optomechanical circuit in which the quantum properties of its motion can be exploited.
Antiferromagnetic skyrmion crystal which is predicted to host topological magnons and chiral magnonic edge states.
A scanning quantum sensor containing a single, scannable spin. For nanoscale imaging, the spin (a “Nitrogen-Vacancy” center in diamond) is held within 10 nm from the lower extremity of the single-crstalline, all-diamond scanning probe (blue). Scalar = 2 um.
Multistage Zeeman decelerators are used to slow down beams of paramagnetic atoms and molecules. These decelerated beams are well suited for high-resolution spectroscopic experiments.
Quantum sensing with a nanoparticle levitated in the focus of a laser beam in high vacuum. The system is ideally decoupled from the environment, provides a high degree of control and ultrahigh sensitivity.
Simulated result of a quantum-state-tomograph experiment. Quantum tomography helps to translate between measurement data and theoretical descriptions of quantum experiments.
The picture shows how an untrusted quantum device (black box) can be compared to an ideal device (white cylinder) with the help of well defined mathematical tools (maps represented by red dotted squares). The aim of these research activities is to certify the proper functioning of arbitrary quantum technologies independently of the imperfections of the actual implementation.
A suspended graphene flake is contacted with palladium (yellow) and ferromagetic permalloy (blue). This device is used to measure the injection of spins into ultraclean graphene.
Illustration of a quantum network combining semiconductor quantum dot single photon sources and atomic quantum memories. Such networks are of interest for quantum communication, simulation and computing.
The electron-microscope image of an ant gives a size reference for a superconducting quantum bit and its control circuitry. Even on such a macroscopic scale, these electronic circuits exhibit quantum-physical properties that can be exploited for quantum information processing.
Ion trap for molecular quantum logic.
An electron-microscope image of nanoparticles containing a crystalline, optically active core and an amorphous coating that changes the way the core interacts with the environment. These nanoparticles are of interest for LED or solar cell applications.
As singe electrons are filled into the double well of a semiconductor nanostructure, the energy levels shift in a characteristic manner. Precise control over the electronic states of such structures is a prerequisite for their use in quantum information processing.
An illustration of a 2D array of coupled photonic waveguides that realizes a 2D topological pump, and unravels the boundary phenomena of the 4D quantum Hall effect.
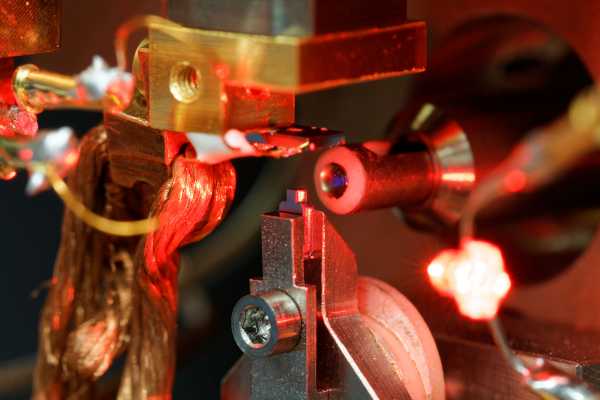
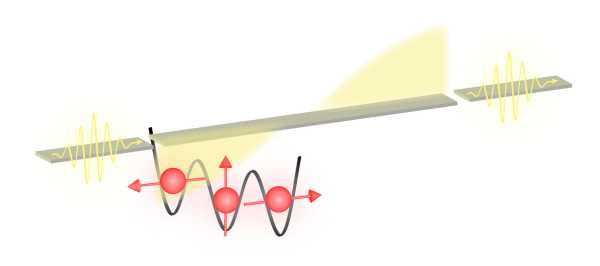
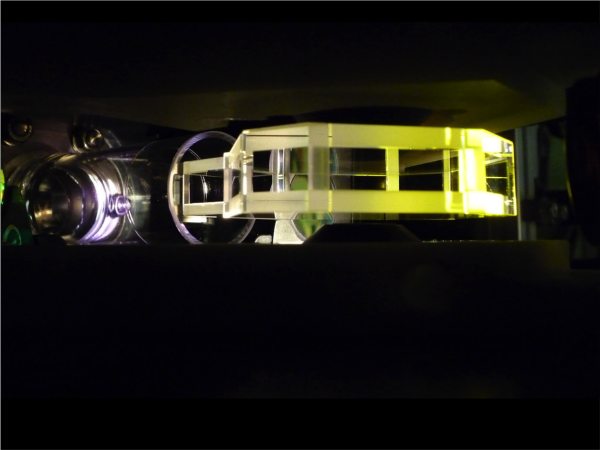
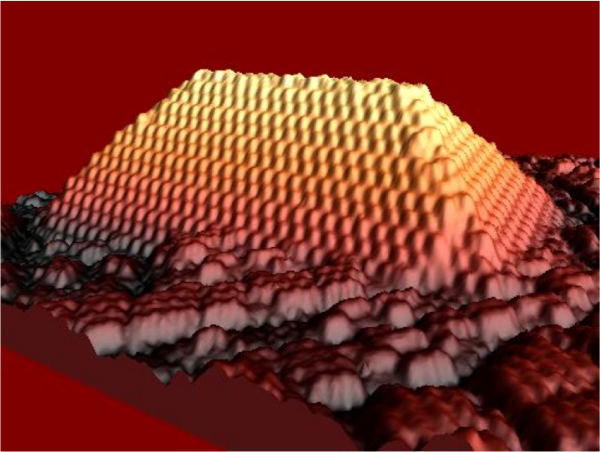
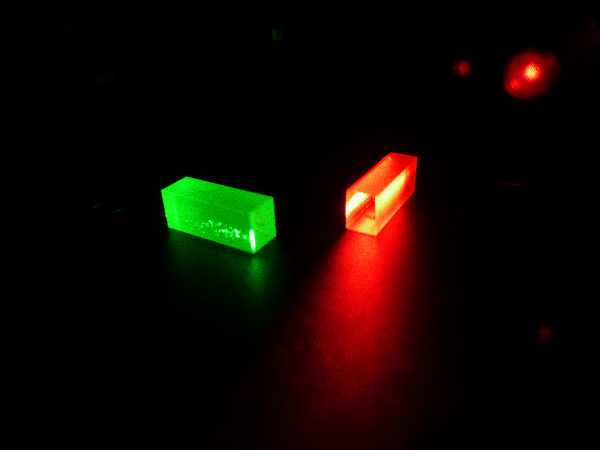
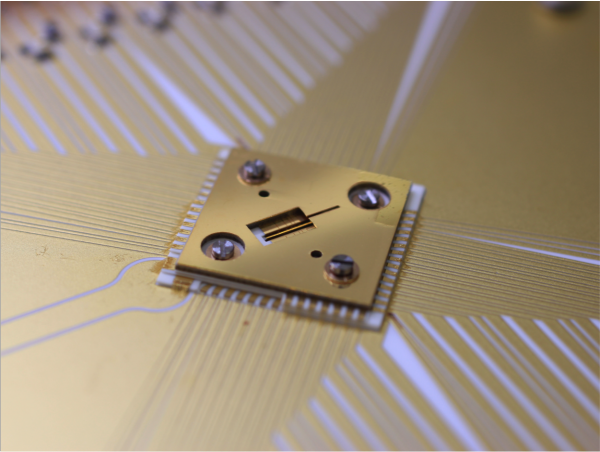
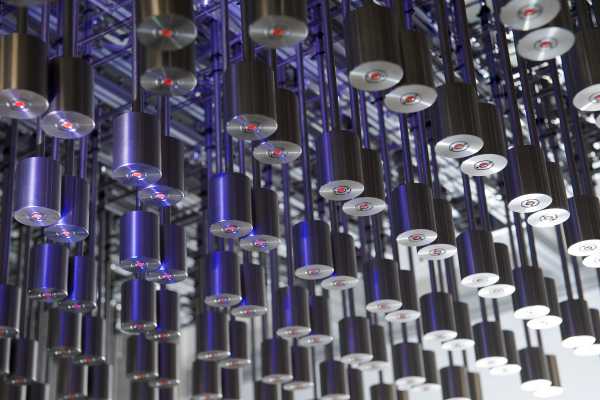
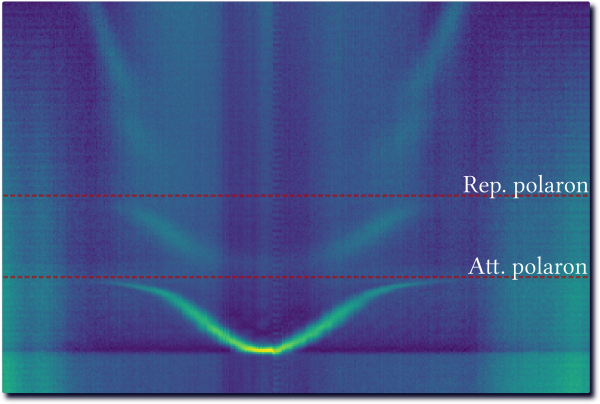
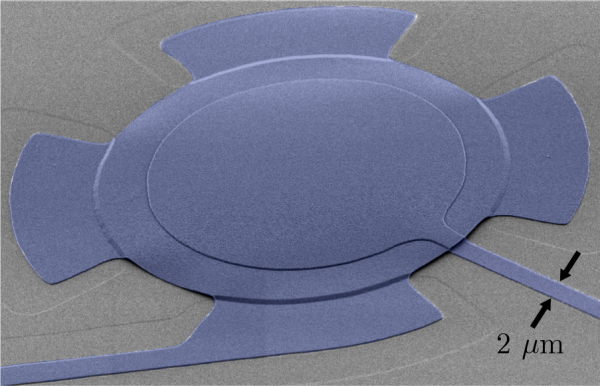
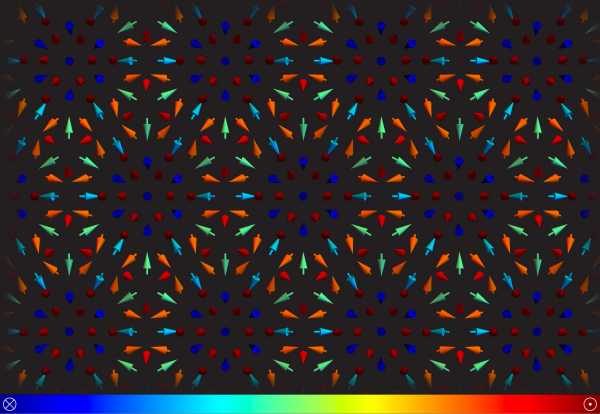
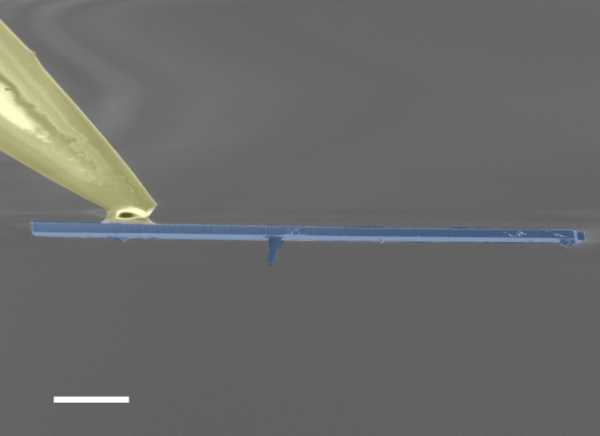
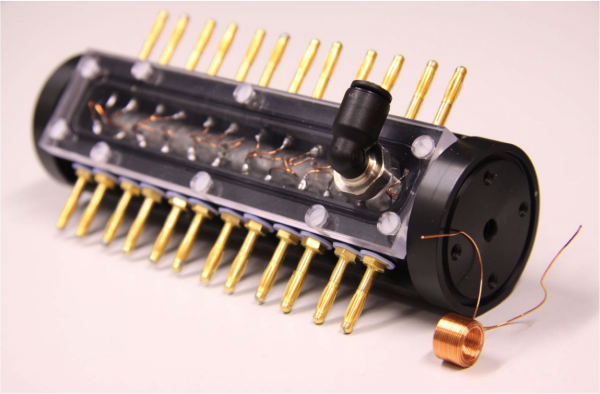
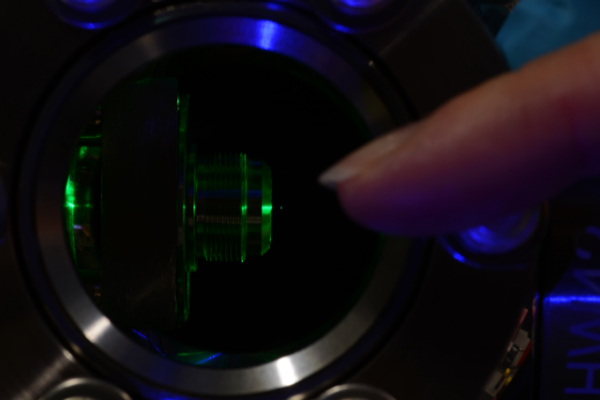
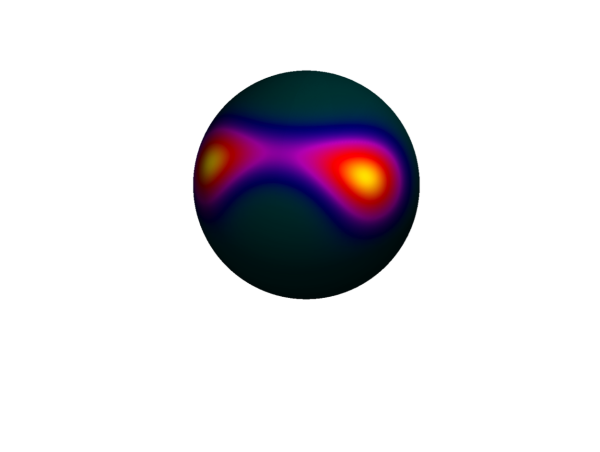
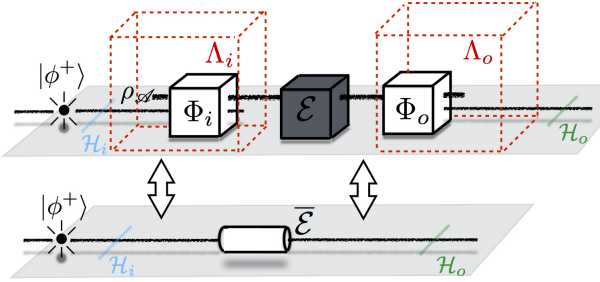
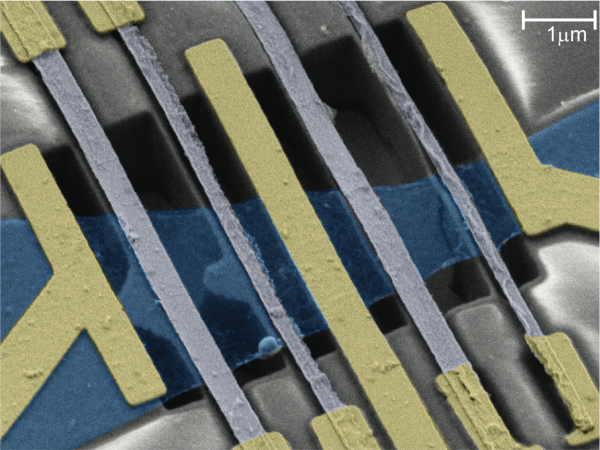
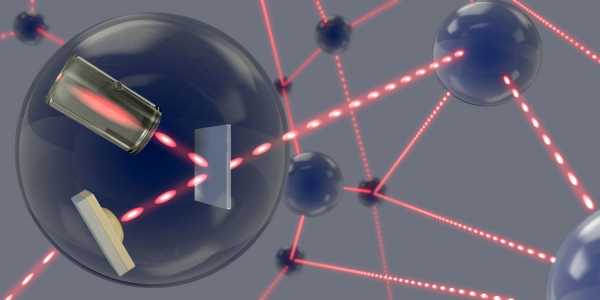
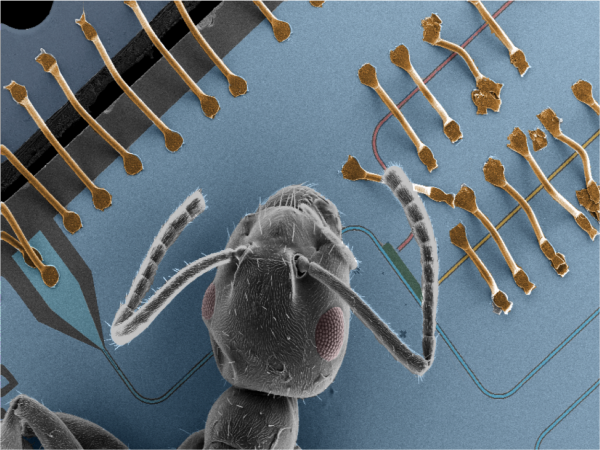
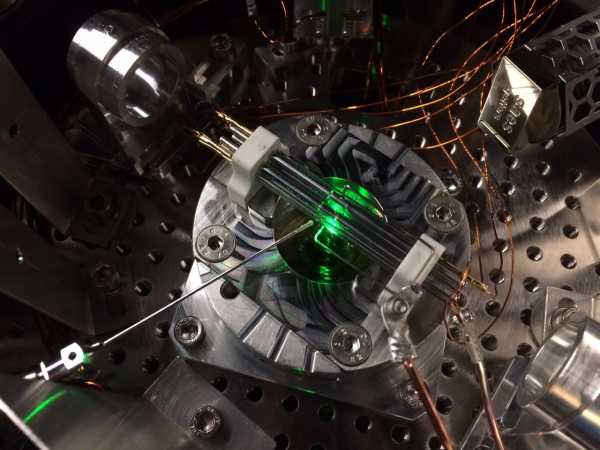
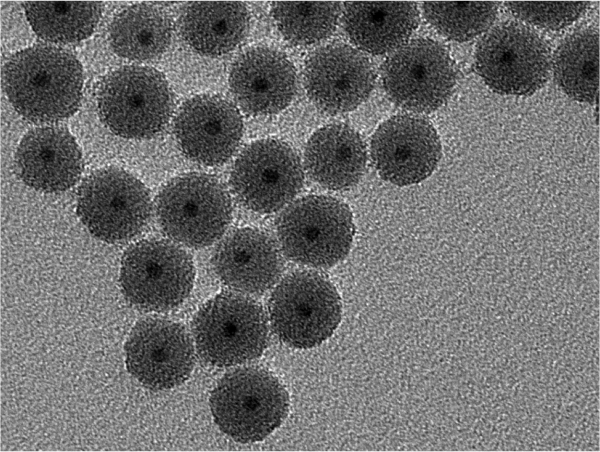
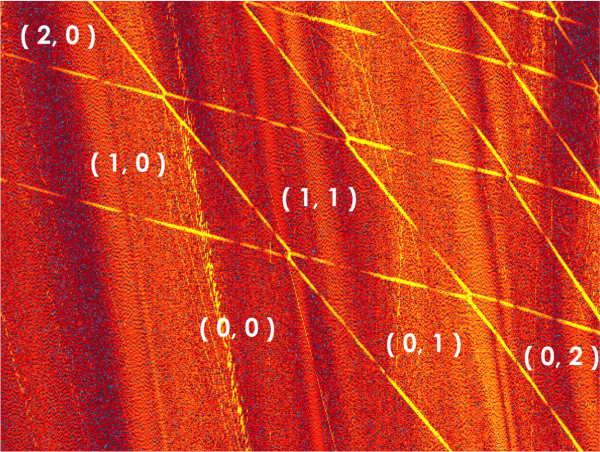
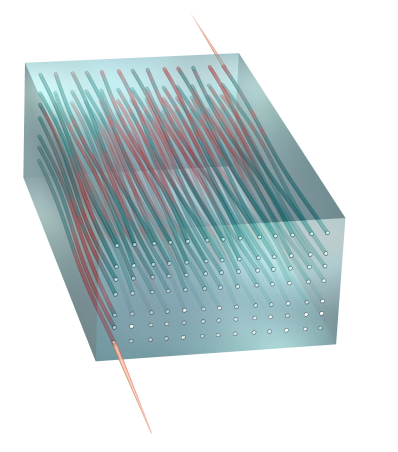